Indonesian island Anak Krakatau has been the site of frequent eruptions. One, in 1883, was catastrophic, killing over 36,000 people, mostly due to the tsunamis it generated. A 2018 eruption caused a tsunami that resulted in 420 deaths and extensive destruction and displacement.
Tsunamis. Volcanoes. Hurricanes. Earthquakes.
Such disasters are the stuff of our greatest fears and, at the same time, our intense fascination. When they strike, we are reminded that, in the words of celebrity astrophysicist Neil deGrasse Tyson, “Here on Earth, we’re still at the mercy of nature.” That’s why URI oceanographers, engineers, and geoscientists are at the forefront of research focused on predicting and mitigating damage from the “next big one.”
By Todd McLeish
When an eruption triggered a portion of Krakatau, a volcano on the island of Anak Krakatau between Java and Sumatra, to collapse into the sea just before Christmas 2018, it caused a tsunami that struck the coast of Indonesia with waves up to 36 feet high, killing 420 people and displacing 40,000 more from their homes. It also caused URI faculty members—tsunami expert Stephan Grilli, professor of ocean engineering, and volcanologist Steven Carey, Ph.D. ’83, emeritus professor of oceanography—to spring into action.
Using tide gauges, satellite images, and photographs, Grilli created a computer model showing how part of the southwest side of the volcano slid into the water and generated the tsunami, while Carey and colleagues from the United Kingdom estimated the volume of the collapse based on radar images. This data allowed them to test ideas and validate other models to better prepare the United States for future tsunamis.
“We have seismic sources, volcanic islands, and underwater landslides in the Atlantic and Pacific oceans that could cause tsunamis to hit our coastlines,” says Grilli. “So we’re creating tsunami simulations and maps to show the potential inundation we could face.”
Grilli and Carey are among more than a half-dozen URI faculty members who pay close attention to natural disasters around the world and conduct research aimed at better understanding these phenomena. Their efforts, and those of numerous alumni, are also helping to forecast and prepare for future disasters.
URI researchers Annette and Stephan Grilli (above) work on models, maps, and assessments to help prepare for potential tsunami risks.
Targeting Tsunamis
A major tsunami that struck Papua New Guinea in 1998 was what Grilli calls his “eye-opener.” Since then, he has studied tsunamis around the globe, historic and modern, and he co-led the first international expedition to investigate the cause of the 2004 Indian Ocean tsunami that killed 230,000 people. He says tsunamis are usually triggered by earthquakes that cause the seafloor to shift upward, forcing the ocean surface to move up and creating a tsunami wave. Underwater landslides also commonly cause tsunamis. “You don’t need a big earthquake to trigger a landslide,” says Grilli, who collaborates with his wife, associate research professor Annette Grilli, on many of his projects. “If you have sediment destabilized on the continental shelf, a little bit of seismicity could cause it to slide down the shelf slope.”
Although the East Coast isn’t at high risk for a tsunami, the Grillis work with the National Tsunami Hazard Mitigation Program to model potential tsunamis in the region and create inundation maps so local emergency management agencies can better prepare for all possibilities. (Juan Horrillo, M.S. ’97, associate professor of ocean engineering at Texas A&M University, is creating similar models and maps for the Gulf of Mexico.) They also conducted tsunami hazard assessments of U.S. nuclear power plants following the tsunami that caused a meltdown of the Fukushima Daiichi Nuclear Power Plant in Japan.
Annette Grilli’s role in these studies has been to assess the variability of wave impacts on the shoreline based on the tsunami’s source—the movement of the seafloor, the landslide, or whatever else propagated the waves—to quantify local risk. Her interest in shoreline impact complements other work she does modeling the effects of severe storms on coastlines and conducting risk assessments for coastal structures.
“Tsunamis are extreme events, but rare, while severe storms are more frequent—and their frequency is increasing,” she says. “People are still buying real estate along the water, and the risk to those buildings from sea-level rise, storm surge, and wave run-up is growing.”
Mount Vesuvius, near Naples, Italy, seen from the ruins of Pompeii. The taller cone on the left is active, although it hasn’t erupted since 1944. URI volcanologist Steve Carey says an eruption could be devastating because of the number of people living nearby.
Volcanoes—Past and Future
Since volcanic eruptions can play a role in generating tsunamis, Grilli occasionally collaborates with Carey, who has been conducting research on volcanoes since his days as a doctoral student at the Graduate School of Oceanography in the 1970s and ’80s. When Mount St. Helens erupted in 1980, Carey and Haraldur Sigurdsson, emeritus professor of oceanography, hiked into the volcano, and Carey became mesmerized by the explosive nature of the eruption and the total destruction it caused. Now he is focused on forensic volcanology.
“I look at the geologic record and the deposits from older eruptions and try to reconstruct the source characteristics of the eruption,” says Carey. “To do that, we study recent volcanoes and the kinds of deposits they produce and the kinds of eruptive processes that take place.”
About 500 volcanoes on land have been active during modern times, mostly around the Ring of Fire, which encircles the Pacific Ocean. A handful of eruptions may be happening at any one time, each usually lasting for weeks or months. Two kinds of eruptions typically occur, according to Carey. Explosive eruptions generate large columns of volcanic gas and ash that can disrupt air travel and cause temporary climate cooling for a year or more. They also generate pyroclastic flows that can move at very high speeds. Effusive eruptions, on the other hand, generate slow-moving lava flows that pose little risk to humans and no significant gas or ash.
Carey says that about 70 percent of volcanic activity occurs underwater, so the eruptions are seldom seen or reported. He has studied underwater volcanoes around the world, including the Kolumbo volcano in the Aegean Sea and the Kick ’em Jenny volcano in the West Indies. His latest project focuses on an underwater volcano off Baja California that produces a little-studied “balloon eruption,” in which blocks of basaltic rock containing large pockets of gas float to the surface.
His most exciting detective work has been at the site of the 79 A.D. eruption of Mount Vesuvius in Italy, where his investigations with Sigurdsson helped unearth details about the characteristics of the eruption that buried the Roman cities of Pompeii and Herculaneum. That’s also the volcano Carey is most concerned about. It has erupted more than 50 times since it wrought devastation on Pompeii, but the last time was in 1944, and he says that the longer it sits idle, the larger the next eruption is likely to be. And because more than a million people live nearby, another big eruption could result in a terrible loss of life.
“It’s actually advantageous for volcanoes to erupt frequently because it lets them blow off steam,” Carey says. “If there’s a long dormancy period, then a large volume of magma can accumulate, making the next eruption even larger. The work we did on the 79 A.D. eruption provided insights about what areas are potentially going to be impacted by a future eruption of Vesuvius. It’s a sobering fact that a million people could be affected.”
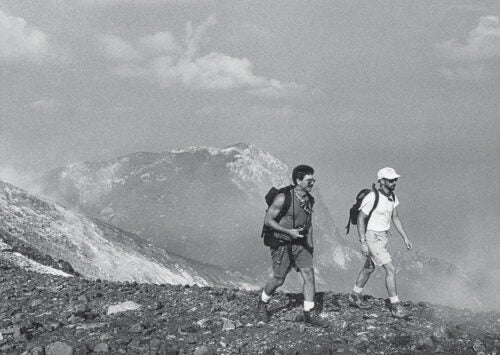
One of Carey’s former graduate students, Charles Mandeville ’81, Ph.D. ’95, leads the Volcano Hazards Program of the U.S. Geological Survey, where he manages nationwide efforts to forecast hazardous volcanic activity and create early warning systems to help land managers and emergency responders minimize potential volcanic impacts. The program operates five volcano observatories with a staff of 200 scientists and a $30 million annual budget.
“We also have the equivalent of a sixth volcano observatory that responds to volcanic crises in developing countries throughout the world,” Mandeville says. Congress recently reauthorized the National Volcano Early Warning System, “so we will be busy for the next five years closing monitoring gaps on very-high-threat and high-threat volcanoes in the U.S. and its territories and augmenting volcano monitoring networks where they need to be modernized,” he added.
Whether a particular volcanic system will produce a dangerous explosive eruption or a less-worrisome effusive eruption is one of the underlying issues in URI oceanography professor Katherine Kelley’s research. She aims to understand why the solid matter inside the planet sometimes melts to create the liquid magma that rises up to the surface and erupts at volcanoes.
“One of the conditions that is extremely favorable to the creation of magma happens when the inside of the Earth gets wet,” she says. “When water from the ocean layer is brought down into the Earth’s interior through plate tectonics, it causes the interior rocks to melt in a chemical reaction similar to when you add salt to icy roads in winter.” And the more water the magma contains, the more explosive the eruption.
By studying the magma ejected from volcanoes—as she is now doing with volcanoes in the Aleutian Islands of Alaska and off the coast of Mexico—Kelley can reconstruct the eruptive process and calculate the water content of the magma before it erupted.
“By knowing what a certain volcanic system might have done in the past, it helps us look forward to what kind of behavior we can expect in the future,” she says.
Hurricane Sandy, shown in this NASA image, was the deadliest and most destructive of the 2012 Atlantic storm season, killing 233 people and inflicting $70 billion in damage. URI professor Isaac Ginis uses Hurricane Sandy as a model to simulate hurricane impacts on Rhode Island in his efforts to better predict damage to specific locations, ultimately aiming to mitigate damage and fatalities.
Hurricane Warning
While it is difficult to predict exactly when and where a major volcanic eruption will take place, it is getting easier to forecast hurricanes, thanks in part to the modeling efforts of URI oceanography professor Isaac Ginis. Recent improvements in hurricane forecasting are due largely to a better understanding of the role the ocean plays in determining the path and intensity of hurricanes, which has been the topic of Ginis’ research for more than 25 years.
“Hurricanes draw energy from the ocean, and if the ocean temperature is higher, then hurricanes become more intense,” he says, noting that as the changing climate warms the ocean, hurricanes will continue to grow in intensity. “We’re also seeing that hurricanes are intensifying more rapidly, going from a Category 1 to a Category 3 or 4 within a day or two. And that’s a big concern for forecasters who want to issue hurricane warnings in advance of landfall.”
Ginis says hurricanes are now producing more rainfall than they used to, as well. “Rain comes from evaporation from the ocean, and the evaporation rate is a function of ocean temperature,” he says. “The warmer the water, the more evaporation. So hurricanes are getting more moisture from the ocean, and because of the higher air temperatures, they can hold more moisture in the atmosphere and produce more rain. That will continue as global warming continues.”
Ginis’ hurricane models, which incorporate these complex interactions between the ocean, atmosphere, and storms, have been coupled with several of the models used by the National Hurricane Center to predict hurricanes and typhoons in the Atlantic and Pacific. His models are also being used by the U.S. Navy to predict storm intensity wherever Navy ships travel.
In recent years, Ginis has also worked with the Department of Homeland Security to improve predictions of the hazards that hurricanes can cause to infrastructure when they make landfall. “One of the major challenges in predicting hazards is that you need to make predictions of wind and flooding at specific geographical locations, like bridges or hospitals,” he says. “General weather prediction models aren’t specific enough for helping emergency management agencies make decisions.”
Many of Ginis’ former graduate students are now applying this research in a variety of contexts. At AIR Worldwide, for instance, Richard Yablonsky, Ph.D. ’09, Michael Bueti, Ph.D. ’14, and Austen Blair, Ph.D. ’16, work together to develop analytical tools that insurance companies use to assess their potential risk from hurricanes and other natural disasters.
“Our role is focused primarily on the storm surge that’s produced by hurricanes and typhoons in the U.S. and winter storms in the U.K.,” says Yablonsky, a certified extreme event modeler and former recreational tornado chaser. “We’re building computer models to simulate those types of events and give guidance as to what the risk is to our customers. We give them the best data possible so they can make more informed decisions about what properties to insure and how much to charge.”
An aerial view of the San Andreas Fault in California’s Sierra Madre Mountains. The fault is the boundary between the Pacific and the North American tectonic plates.
URI researchers Yang Shen (above) and Matt Wei study underwater faults, which produce earthquakes more frequently than faults on land.
The Holy Grail of Earthquake Studies: Prediction
In an active year, there may be more than 20 tropical storms or hurricanes in the Atlantic and far fewer in the Pacific and Indian oceans. But there are likely many thousands of earthquakes each year, most barely detectable by even the most sensitive instruments and usually caused by shifts in the Earth’s tectonic plates. Predicting when an earthquake will occur is what URI oceanography professor Yang Shen calls the holy grail of earthquake studies.
“We can’t predict exactly when it’s going to happen, but we have a good idea for intermediate-term or long-term predictions,” says Shen. “We can see the probability of a big earthquake occurring in a particular place, but we cannot say which year or which day it’s going to happen.”
Shen has been interested in earthquakes since childhood, when a massive quake in China in 1976 killed 200,000 people. Since joining the URI faculty in 1998, he has studied earthquakes around the world, from Iceland to Easter Island and the Galápagos. In 2018, he deployed seismometers on the ocean floor off Hawaii to record the aftershocks from the eruption of the Kilauea volcano—which has been erupting off and on since 1983—to learn which fault system had been activated.
“We’re still analyzing the data, but using all available data from land-based and offshore seismometers, we detected a lot of earthquakes, an order of magnitude more than were published by the Hawaiian Volcano Observatory,” he says. Using this data, he is now engaged in a project to use machine learning to detect and locate earthquakes faster and more precisely than ever before. This effort should reveal smaller earthquakes and unknown fault lines that could be precursors to major earthquakes.
Among his collaborators is Matt Wei, URI assistant professor of oceanography, who studies earthquake cycles—the interval, magnitude, and location of earthquakes that repeatedly rupture a particular fault. Because most faults on land produce strong earthquakes hundreds or thousands of years apart, an accurate record of the cycles on these earthquakes is not available, making it difficult to understand earthquake behavior. But some faults beneath the ocean can generate magnitude 6 earthquakes every three to five years, which allows scientists like Wei to study the seismic cycles at these faults to gather information that may detect patterns in earthquake occurrence.
Using data from seismometers around the world, Wei is building computer models that show the interactions between earthquake cycles in ocean faults, which can then be applied to better understand the cycles and interactions in faults on land, like the San Andreas Fault in Southern California, which is overdue for a large earthquake.
Wei is also studying a phenomenon called slow earthquakes. “They have the same physics as earthquakes, but they happen very slowly so they don’t generate earth shaking and don’t damage buildings,” he says. “But they do interact with large earthquakes, and we think slow earthquakes may trigger large earthquakes. We may be able to use them to predict large earthquakes.
“Slow earthquakes also release stress on faults,” Wei adds. “If detected offshore in subduction zones, we expect future earthquakes to be smaller than previously thought.”
Detecting slow earthquakes that occur beneath the ocean is especially challenging because it requires measuring the deformation of the seafloor down to the millimeter scale. Most scientists use a pressure sensor placed on the ocean bottom to detect movements of the seafloor, but it’s difficult to separate tectonic movements from the changing ocean pressures. So Wei and Yang are using machine learning methods to better detect slow earthquakes from the seafloor pressure data, which may help in hazard assessments.
Not only can the seismic waves produced by earthquakes tell scientists about the characteristics of a particular earthquake, they can also be used to learn about other features of the Earth’s structure. Brian Savage, associate professor of geosciences, partnered with Shen in using seismic data to learn why the oceanic crust in an area of the Western Pacific called the Ontong Java Plateau is about 35 kilometers thick, four or five times thicker than typical oceanic crust around the globe. He recorded the speed of seismic waves traveling through the Earth from earthquakes to create an image of the structure below the plateau.
“We’re trying to understand why this large plateau was built, why it’s so big, and why it’s so weird compared to others,” Savage says.
Savage uses a similar methodology to study how the Earth’s tectonic plates look under California or the East Coast to better understand the processes that are shaping the planet. Seismic data from the Middle East has even helped him develop models to monitor underground nuclear explosions and differentiate them from earthquakes.
“Some countries want to hide the fact that they’re testing nuclear devices, but those events have particular characteristics that look like explosions rather than earthquakes,” Savage says. “When we measure them, there are uncertainties in how the waves propagate. Getting better estimates of how those waves move from one place to another helps us characterize those events better.”
Aerial view from an Army search-and-rescue mission shows damage from Hurricane Sandy to the New Jersey coast in 2012.
What Defines a Disaster?
Regardless of whether it’s an earthquake, tsunami, volcano, hurricane, or climate change, what defines a disaster is its impact on people.
“The difference between a disaster and an interesting phenomenon is how it affects human life,” says Bonnie Epstein, Ph.D. ’99, who has taught a class on natural disasters at the Rhode Island School of Design for 11 years. “A flood where three people lose their house, that’s a shame; but if 1,200 people lose their homes, that’s more like a disaster. We measure it by the cost of what was lost, or by loss of life.”
Much of her class focuses on how human-built structures can either mitigate disasters or make them worse. Flooding from hurricanes, for instance, is often worse in areas where an abundance of pavement causes rainfall to quickly run into rivers rather than be absorbed into the soil and wetlands.
Among her more esoteric recommendations for preparing communities for natural disasters is to tap into community memories from previous disasters. One reason why the 2010 earthquake in Haiti was so destructive, she says, is that there hadn’t been an earthquake there since the 1700s, so there was little memory that such an event could happen and no memory of what residents should do. A more powerful earthquake in Chile the same year had a lesser impact because communities there regularly undergo earthquake drills and other preparations.
“That’s why I’m a fan of public memorials to certain disasters, like the plaques noting the high-water mark from the Hurricane of ’38,” Epstein says. “Even if you don’t discuss disasters with your neighbors, it’s a reminder to pay attention.” •
PHOTOS: GETTY IMAGES; NORA LEWIS; MORN THE GORN; RAGNAR AXELSSON; NASA, MODIS/ LANCE; CAROL M. HIGHSMITH; COURTESY GSO; MARK C. OLSEN
A great article about about research by students and faculty at the GSO.